Case Study: Emergent 100GigE High-Speed Camera Enables Innovative Biomedical Diagnosis
Researchers at Vrije Universiteit Amsterdam are pioneering a novel imaging technique known as phototransient holographic imaging, which provides unprecedented insights into living cells. Central to this advancement is the HZ-21000-G 100GigE camera from Emergent Vision Technologies.
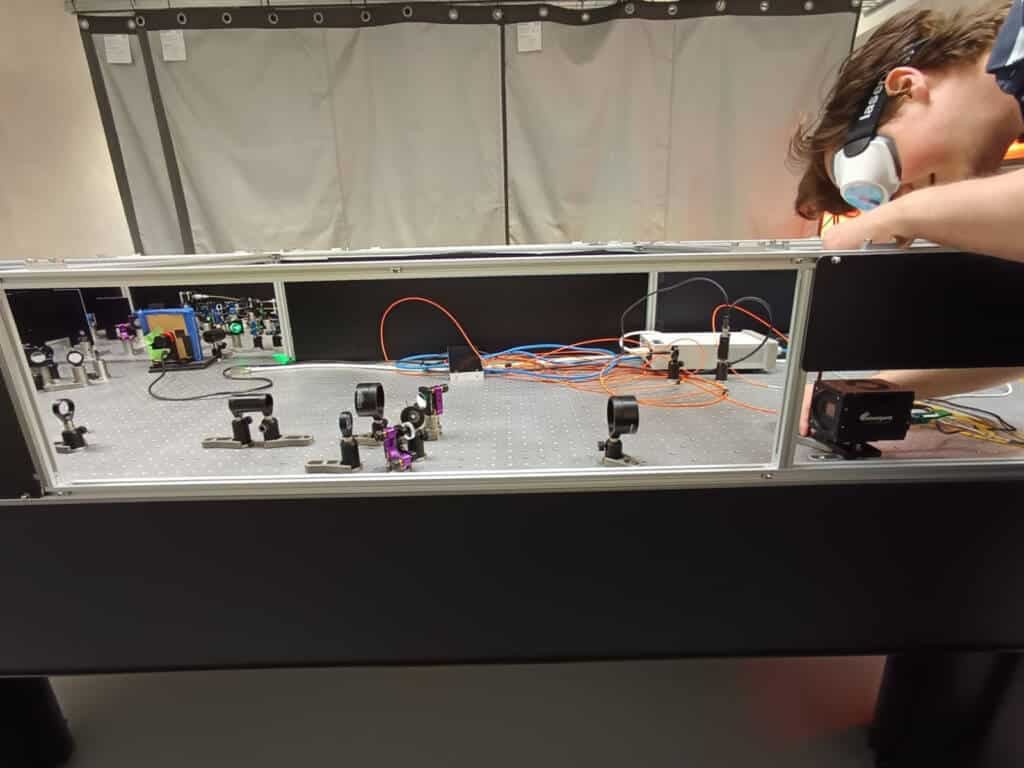
Daan Wolters adjusts the Emergent HZ-21000-G 100GigE high-speed camera that is fundamental to the lab’s work with phototransient holography.
Expanding the frontiers of medical imaging with 100GigE technology
100GigE cameras are revolutionizing medical imaging by capturing highly detailed images at ultra-high frame rates. Their ability to deliver high-speed imaging with minimal CPU overhead and expansive fields of view is unlocking new diagnostic capabilities in advanced microscopy.
At Vrije Universiteit Amsterdam, Ph.D. candidate Daan Wolters is advancing phototransient holographic imaging, a technology originally developed in the Matzlab under the guidance of Matz Liebel. This technique leverages high-speed imaging and stroboscopic excitation to enhance the analysis of potentially cancerous cells. Wolters’ research focuses on demonstrating its applicability to biological samples, thereby pushing the boundaries of biomedical diagnostics.
The Matzlab team specializes in biomedical imaging, disease diagnosis, and pharmaceutical quality control. Their application of phototransient holography to histopathology aims to facilitate more accurate and expedited diagnostics.
Redefining Cancer Tissue Analysis
Current cancer diagnostics rely on traditional histological methods: a surgeon extracts a tissue sample, which is then thinly sliced, stained, and examined under a microscope. These images are analyzed to determine whether the tissue is cancerous and its stage of development.
“This method, although considered the gold standard, has remained largely unchanged for over half a century,” notes Liebel.
In contrast, the VU Amsterdam team’s approach enhances conventional diagnostics by extracting richer, quantitative imaging data from tissue samples. Their phototransient holography technique, powered by the Emergent HZ-21000-G 100GigE high-speed camera, captures transient dynamics and photoinduced signals, enabling deeper insights into cellular composition and function.
“If you consider that biological organisms are primarily composed of sugars, fats, nucleic acids like DNA, proteins, and water, our goal is to quantify these fundamental building blocks,” explains Liebel. “This is particularly relevant in cancer research, where altered metabolic activity is a hallmark of malignancy. By visualizing these functional differences, we gain crucial diagnostic insights.”
Phototransient holographic imaging builds on quantitative phase imaging (shown here), a microscopy technique that measures light phase shifts as it passes through transparent or semitransparent samples.
How phototransient holography works
At its core, phototransient holography builds upon quantitative phase imaging, a microscopy technique that measures light phase shifts as it passes through transparent or semi-transparent samples. This enables precise assessment of cellular biomass by analyzing light propagation.
Instead of traditional staining methods, such as hematoxylin and eosin (H&E) staining, phototransient holography relies on light interaction with the sample to visualize its molecular composition. The technique integrates holographic imaging, which captures both light intensity and phase, providing researchers with detailed information about sample thickness and composition.
“Taking this a step further, we can spatially resolve cellular mass distribution,” says Liebel. “Standard quantitative phase imaging provides mass information, but it does not reveal chemical composition. Phototransient holography addresses this limitation.”
By directing infrared (IR) or near-infrared light onto the sample, specific biomolecules—such as proteins, lipids, or nucleic acids—selectively absorb the energy. This localized absorption induces a temperature change, which alters the phase shift detected through holography.
“We observe a phase retardation effect, where temperature fluctuations become detectable via holography,” Liebel explains. “By subtracting images taken before and after IR exposure, we generate molecular-specific contrast images that reveal protein distribution. Adjusting the excitation wavelengths allows us to differentiate between various biomolecular components, such as lipids.”
Stroboscopic excitation, combined with high-speed imaging, further enhances image quality, ensuring precise and reproducible measurements.
The role of the 100GigE camera
The Matzlab team employs the Emergent HZ-21000-G 100GigE camera due to its exceptional speed and large field of view. This enables them to capture extensive sample areas—such as entire tissue slides—in rapid succession while switching light sources on and off.
Speed is particularly critical for imaging living samples. The rapid acquisition of stroboscopic images ensures that motion artifacts are minimized, maintaining consistency between heated and non-heated samples.
Compared to conventional IR absorption imaging, the phototransient approach significantly enhances spatial resolution, unveiling intricate cellular details rather than producing indistinct, blurred structures. Furthermore, the non-invasive nature of this technique minimizes sample excitation and photodamage, an essential consideration for future clinical applications.
“Our goal is to capture images with minimal laser exposure while ensuring high signal-to-noise ratios,” Liebel states. “A high-speed camera enables us to collect a substantial number of photons per frame without overheating or damaging the sample.”
A major advantage of this transient read-out method is its ability to perform large field-of-view observations with superior signal levels.
“In an ideal scenario, we would acquire identical images—one with heating and one without—so that any observed differences are purely biochemical,” Liebel explains. “The faster our imaging, the greater the likelihood of capturing an unchanged sample, which is even more crucial when working with living tissue.”
Dennis van de Lockand (left) and Daan Wolters, who work on the phototransient holographic imaging research.
Real-Time Hologram Processing with GPU Acceleration
Beyond its imaging speed, the Emergent camera also facilitates real-time hologram processing on a graphics processing unit (GPU) in the computer to which the camera is routed, an essential feature for computational efficiency.
“This is invaluable for us, as hologram processing is computationally intensive and requires extensive Fourier transformations,” says Liebel. “With the Emergent camera, we acquire images directly on the GPU, extract phase information, compute mass differences between heated and non-heated samples, apply averaging, and then stream only the reduced data to the computer.”
In contrast, traditional imaging workflows often involve streaming raw data, which can lead to storage bottlenecks and limit continuous acquisition. By processing data on the GPU, the Matzlab team optimizes efficiency and maximizes throughput.
Optimized Light Collection Per Pixel
In addition to GPU capabilities, another decisive factor in selecting the Emergent camera was its superior light collection efficiency.
“The 2 × 2 pixel binning hardware option allows us to double the frame rate while quadrupling the light per pixel,” notes Liebel. “Instead of utilizing the full 21-megapixel capacity, we operate at approximately 4 to 4.5 megapixels but at a significantly higher frame rate—around 1,600 frames per second.”
This optimization aligns the camera’s resolution with the optical specifications of a microscope, ensuring optimal imaging performance.
Toward Clinical Application
Over the past few years, the Matzlab team has conducted proof-of-concept experiments, integrating holographic all-optical lock-in detection and demonstrating the technique’s spectroscopic and sensing capabilities. Their research includes phototransient 3D particle tracking and time-resolved imaging of freely moving samples.
The next phase of development focuses on refining the technique for biomedical applications, including the identification of bacteria, cells, and tissue types. The researchers aim to transition from proof-of-principle experiments to clinical implementation within the next two to three years.
Looking ahead, Liebel envisions future cameras with even greater speed and sensitivity, enabling detection of subtler biological variations.
“The ultimate goal is to develop phototransient holography for direct, in vivo patient imaging,” he concludes. “Although we are still far from that point, this technology has the potential to redefine histopathology and revolutionize medical diagnostics.”